How Biocombinatorial Junction Engineering Will Reshape Biofabrication in 2025: The Next Leap in Synthetic Biology and Advanced Material Science Revealed
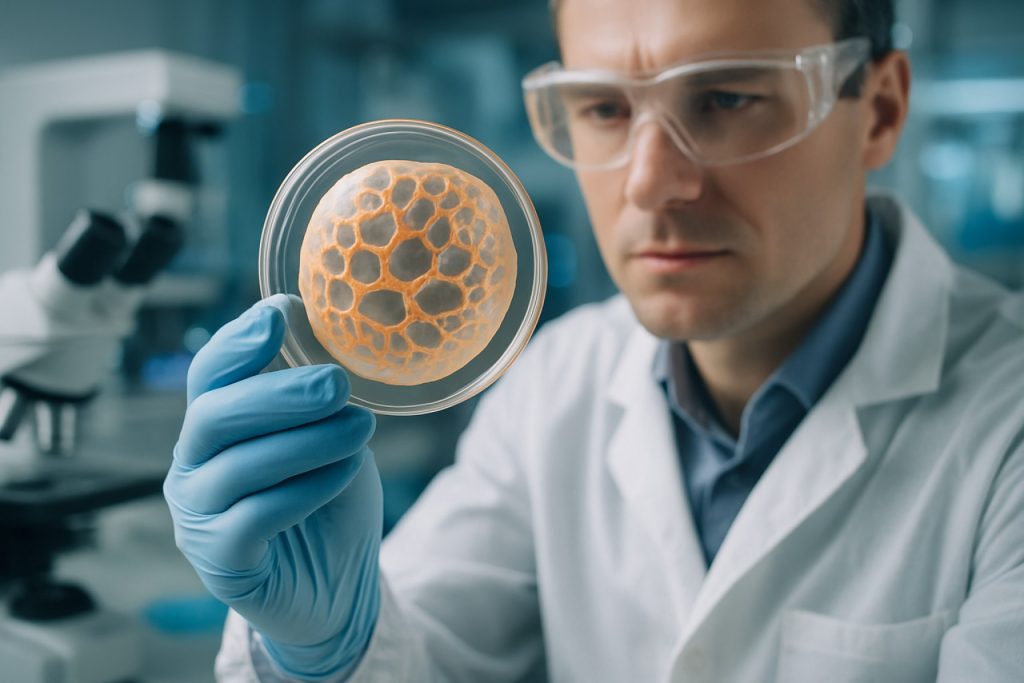
Biocombinatorial Junction Engineering: 2025’s Billion-Dollar Breakthrough & What’s Next
Table of Contents
- Executive Summary: Key Trends and Market Drivers
- Biocombinatorial Junction Engineering Explained: Fundamentals & Definitions
- 2025 Market Landscape: Industry Size and Growth Projections
- Breakthrough Technologies: Innovations Powering the Sector
- Key Players & Strategic Collaborations (Source: syntheticbiology.org, ginkgobioworks.com)
- Applications Across Sectors: From Biomedicine to Smart Materials
- Regulatory Environment and Emerging Standards (Source: isaaa.org, syntheticbiology.org)
- Investment, Funding, and M&A Activity in 2025
- Challenges & Risks: Technical, Ethical, and Commercial Barriers
- 2025–2030 Outlook: Future Opportunities and Transformational Trends
- Sources & References
Executive Summary: Key Trends and Market Drivers
Biocombinatorial junction engineering—leveraging combinatorial biology and synthetic engineering to optimize molecular interfaces—has rapidly emerged as a transformative approach in advanced materials and biotechnology sectors. The field is characterized by the rational design and evolution of junctions in proteins, DNA, or hybrid biomaterials to achieve novel functionalities, enhanced stability, and tailored interactions. As of 2025, several key trends and drivers are catalyzing innovation and market adoption.
- Convergence of AI and High-Throughput Screening: The integration of machine learning algorithms with high-throughput experimental platforms has allowed for the rapid identification and optimization of biojunctions. Companies like Amyris and Ginkgo Bioworks are utilizing automated strain engineering and data-driven design to accelerate the development of custom biomaterials and enzymes.
- Expansion in Synthetic Biology Toolkits: Advances in gene synthesis, modular cloning, and CRISPR-based editing are enabling unprecedented control over junction design. Tool providers such as Integrated DNA Technologies (IDT) and Twist Bioscience deliver critical components for constructing and screening vast libraries of biomolecular junctions.
- Industrial and Biomedical Applications: Engineered biojunctions are being applied in diverse sectors, from sustainable chemicals to therapeutics. For instance, Novonesis (formerly Novozymes) is optimizing enzyme junctions for industrial catalysis, while Sangamo Therapeutics advances gene editing platforms that rely on precise protein-DNA interfaces.
- Scalability and Commercialization: The ability to scale from laboratory discovery to industrial manufacturing is a critical driver. Companies are investing in biomanufacturing infrastructure, as seen with Lonza’s expansion of cell and gene therapy production, supporting the translation of engineered junctions into commercial products.
Looking ahead to the next few years, the sector is expected to see further convergence with digital biology and automation, as well as increased demand for sustainable and high-performance bio-based materials. Collaborations between technology suppliers, biomanufacturers, and end users will be pivotal in driving both market growth and the emergence of new application domains for biocombinatorial junction engineering.
Biocombinatorial Junction Engineering Explained: Fundamentals & Definitions
Biocombinatorial Junction Engineering is an emerging discipline at the intersection of synthetic biology, materials science, and molecular engineering, focusing on the deliberate design and assembly of biological, hybrid, or bio-inspired interfaces—often called “junctions.” These junctions function as controllable sites of interaction or communication between distinct biological or bioengineered components, ranging from proteins and nucleic acids to living cells and synthetic materials. The core concept involves leveraging combinatorial strategies—systematically varying the sequence, structure, or environmental parameters—to optimize the properties and performance of these interfaces for targeted applications in areas such as biosensing, tissue engineering, and regenerative medicine.
At the fundamental level, junction engineering entails the precise arrangement of molecular or cellular building blocks to create interface regions with bespoke properties. For example, in protein engineering, combinatorial libraries of surface residues are routinely generated and screened to identify variants that maximize binding affinity or selectivity at protein-protein or protein-material junctions. Recent advances in high-throughput screening and microfluidic sorting, as exemplified by platforms developed by Twist Bioscience and Synthego, have dramatically accelerated the ability to explore vast combinatorial sequence spaces and rapidly iterate on junction designs.
In the context of biomaterials, biocombinatorial junction engineering also encompasses the strategic incorporation of peptides, oligonucleotides, or polysaccharides into surfaces to modulate cell adhesion, signaling, or immune recognition. Companies like Evonik Industries and Cytiva are actively developing biofunctionalized surfaces and scaffolds where combinatorially engineered junctions dictate cellular responses—an approach pivotal to next-generation medical devices and tissue scaffolds.
The field is further defined by the integration of computational modeling, machine learning, and automated laboratory platforms to optimize junction properties in silico before experimental validation. This convergence is embodied in initiatives at organizations such as Ginkgo Bioworks, which utilizes automated foundries and AI-driven design to create new biological junctions with tailored characteristics.
As of 2025, Biocombinatorial Junction Engineering is positioned as a foundational technology underpinning innovations in precision medicine, smart biomaterials, and synthetic cell systems. Its scope is rapidly expanding, with new tools and standards being established by industry leaders, signaling a shift toward modular, programmable interfaces that can be rapidly customized for diverse bioscience and biomedical applications.
2025 Market Landscape: Industry Size and Growth Projections
Biocombinatorial junction engineering, a field at the intersection of synthetic biology, materials science, and molecular engineering, is poised for significant growth as industries seek novel approaches to develop advanced biomaterials and hybrid interfaces. By 2025, the market landscape reflects a surge in demand for biocombinatorial methods to tailor interfacial properties in medical devices, tissue engineering, and biosensors.
Major biotechnology and materials science firms are advancing the use of combinatorial libraries to engineer protein, peptide, and polymer junctions with unprecedented specificity and functionality. For instance, Genentech has expanded its R&D pipelines to include protein interface optimization platforms, while Amgen is integrating biocombinatorial junction engineering in the development of next-generation biologics, optimizing molecular junctions for enhanced drug stability and delivery.
In 2025, the global market size for biocombinatorial junction engineering applications—spanning healthcare, advanced materials, and diagnostics—is estimated to exceed several hundred million USD, with high double-digit compound annual growth rate (CAGR) projections through 2028. This growth is fueled by an uptick in collaborations between academic research institutions and industry leaders, particularly in North America, Europe, and East Asia. Companies such as Thermo Fisher Scientific are investing in modular synthesis and screening platforms to accelerate the translation of biocombinatorial junction discoveries into commercial products.
Emerging start-ups are also contributing to the competitive landscape. For example, Twist Bioscience is leveraging high-throughput DNA synthesis to create combinatorial libraries for junction engineering, while Ginkgo Bioworks is scaling up automated cell programming for the custom design of bio-interfaces. These technological advances are enabling rapid prototyping and commercialization of junction-engineered biomaterials for use in regenerative medicine and smart diagnostics.
Looking ahead, the next few years are expected to bring further integration of artificial intelligence and machine learning to streamline the identification and optimization of functional junctions. Industrial leaders predict that the convergence of automation, data analytics, and biocombinatorial engineering will catalyze the development of new product classes across biomedicine and advanced manufacturing. As regulatory pathways clarify and investment continues to rise, the sector is on track for robust expansion and increasing impact across multiple high-value markets.
Breakthrough Technologies: Innovations Powering the Sector
Biocombinatorial junction engineering is emerging as a transformative force in biotechnology and materials science, leveraging combinatorial biology and advanced molecular design to create novel interfaces between biological and non-biological systems. In 2025, the field has moved beyond proof-of-concept studies, with several key technological advancements and commercialization pathways shaping its trajectory.
A major milestone in 2024 was the demonstration of programmable protein junctions to create self-assembling biomaterials with tunable properties. Companies like Amyris and Ginkgo Bioworks have utilized synthetic biology platforms to screen vast libraries of peptides and proteins, enabling the design of junctions with tailored binding affinities and specificities for targeted applications. These biocombinatorial approaches are now being integrated into the development of hybrid medical devices, where seamless integration between living tissues and synthetic polymers is critical for performance and biocompatibility.
In parallel, the application of automated high-throughput screening systems, such as those offered by Tessella (now part of Cognizant), enables the rapid evaluation of millions of junction variants under physiologically relevant conditions. This capability has accelerated the optimization of interfaces for use in biosensors, tissue engineering scaffolds, and next-generation drug delivery systems.
Academic collaborations with industrial partners have also led to the creation of dynamic junctions that respond to environmental cues, such as pH or temperature. For example, Thermo Fisher Scientific is advancing modular systems that utilize engineered protein domains to modulate cell-material interactions, opening pathways to smart implants and responsive wound dressings.
Looking ahead, the sector anticipates significant growth, driven by the convergence of artificial intelligence, robotics, and combinatorial biochemistry. Automated design-build-test-learn cycles are projected to reduce development timelines and costs, facilitating the translation of biocombinatorial junction innovations into commercial products. Companies like Synthego are expected to play a pivotal role by providing genome engineering tools that underpin custom junction development for therapeutics and diagnostics.
By 2027, industry analysts expect biocombinatorial junction engineering to be central to personalized medicine, advanced prosthetics, and green manufacturing processes. The ongoing integration of these innovations into regulated clinical and industrial pipelines underscores the sector’s robust outlook and its potential to redefine biological-material interfaces across multiple domains.
Key Players & Strategic Collaborations (Source: syntheticbiology.org, ginkgobioworks.com)
Biocombinatorial junction engineering—a frontier within synthetic biology—focuses on the rational design, assembly, and modulation of biomolecular junctions for enhanced functionality in biomanufacturing, therapeutics, and materials science. As of 2025, the sector is defined by a surge of strategic partnerships, licensing agreements, and consortium-based research driven by leading synthetic biology companies and research institutions.
Key players include Ginkgo Bioworks, which continues to expand its cell programming platform, enabling the high-throughput design and optimization of biosynthetic junctions for novel product pathways. In 2024-2025, Ginkgo has announced collaborations with biopharmaceutical and specialty chemical manufacturers to engineer more efficient enzymatic junctions, aiming to enhance yield and selectivity in microbial production systems.
Another significant contributor is Synthetic Biology Engineering Research Center (SynBERC), which, through its ongoing initiatives, supports the development of standardized, modular biological parts. In 2025, SynBERC-affiliated labs are engaged in multi-institutional efforts to establish open-source libraries of junction elements—such as DNA scaffold connectors and protein-protein interfaces—facilitating the rapid prototyping and deployment of biocombinatorial assemblies.
Strategic collaborations are accelerating innovation. For example, Ginkgo Bioworks has entered joint development agreements with agrochemical and pharmaceutical partners to apply junction engineering in the creation of next-generation bioactive compounds. These projects leverage Ginkgo’s automated foundry and organism engineering capabilities, expediting the iterative optimization of junction modules for commercial-scale processes. Similarly, SynBERC’s public-private partnerships have yielded new toolkits and reference standards for junction design, which are being adopted by both academic and industrial stakeholders to streamline regulatory approval and manufacturing scale-up.
Looking ahead, the next few years are expected to see further integration of artificial intelligence and machine learning with combinatorial design platforms. This convergence, championed by organizations like Ginkgo Bioworks, will likely lead to the discovery of novel junction configurations with properties unattainable by traditional methods. As intellectual property portfolios around biocombinatorial junctions expand, licensing and co-development models are anticipated to proliferate, fostering a more collaborative and innovation-driven landscape.
Overall, ongoing investment, open-source initiatives, and strategic alliances among key players are set to accelerate the maturation of biocombinatorial junction engineering, positioning the field for transformative advances in synthetic biology and industrial biotechnology over the next several years.
Applications Across Sectors: From Biomedicine to Smart Materials
Biocombinatorial junction engineering—leveraging combinatorial methods to design and optimize biointerfaces at junctions between materials, cells, or biomolecules—is rapidly advancing in its application across multiple sectors. As of 2025, several industries are harnessing this approach to develop solutions ranging from next-generation biomedical devices to adaptive smart materials.
In the biomedical sector, the precise engineering of tissue-material interfaces has become crucial for the performance of implants, scaffolds, and biosensors. Companies like Evonik Industries are focusing on the development of biopolymer-based junctions that enhance cell adhesion and tissue integration for regenerative medicine. Through biocombinatorial screening, they can rapidly identify optimal polymer blends and surface modifications, promoting better healing outcomes and device longevity.
Similarly, Baxter International Inc. is employing combinatorial approaches to improve biocompatibility in dialysis membranes and blood-contacting medical devices. By engineering the molecular junctions at the membrane interface, they minimize immune reactions and protein fouling, leading to safer and more efficient therapies.
In the realm of smart materials, biocombinatorial junction engineering is enabling the design of adaptive surfaces and interfaces that respond to external stimuli. BASF SE is at the forefront of integrating bioinspired junctions into polymers for self-healing coatings and responsive textiles. Their combinatorial platforms allow the screening of thousands of molecular junction combinations to identify those with optimal mechanical, optical, or chemical responsiveness.
The electronics and biosensing industries are also benefitting from these advances. imec is collaborating with medical device manufacturers to develop bioelectronic interfaces where combinatorial junction engineering enables seamless integration between biological tissues and electronic circuits, improving signal fidelity and device stability.
Looking ahead to the next few years, the outlook for biocombinatorial junction engineering is robust. With the increasing adoption of AI-driven combinatorial design platforms and high-throughput screening technologies, it is expected that both the discovery and commercialization of novel biointerfaces will accelerate. Cross-sector collaborations—such as those between material science leaders and biomedical innovators—will further drive the development of multifunctional products, ranging from smart implants to environmentally adaptive construction materials. As regulatory bodies begin to recognize and standardize methods for evaluating engineered biojunctions, the pathway from laboratory to market is likely to become more streamlined and predictable.
Regulatory Environment and Emerging Standards (Source: isaaa.org, syntheticbiology.org)
The regulatory landscape for biocombinatorial junction engineering—a field at the crossroads of synthetic biology and advanced genetic circuit design—is evolving rapidly as the technology matures and approaches broader industrial adoption. In 2025, the attention of regulators and standards bodies is increasingly focused on ensuring the safety, traceability, and interoperability of genetically engineered constructs, particularly those created using modular, combinatorial approaches.
A cornerstone event in the past year has been the ongoing revision of biosafety guidelines for synthetic biology applications by international bodies such as the International Service for the Acquisition of Agri-biotech Applications (ISAAA). ISAAA has highlighted the need for updated risk assessment frameworks that account for the unique properties of combinatorial genetic junctions, which often recombine genetic elements from multiple species and functional domains. Stakeholder consultations in 2024-2025 are shaping new criteria for environmental release and monitoring, particularly for organisms developed with automated, high-throughput assembly methods.
At the same time, the Synthetic Biology Open Language (SBOL) community, organized through the Synthetic Biology Standards Consortium, has been advancing specifications for representing and sharing biocombinatorial junction designs. In early 2025, the group introduced updates to the SBOL standard to better capture modular interfaces and sequence-level junctions. This update aids regulatory review by improving transparency and reproducibility in the documentation of engineered constructs.
The drive for harmonized standards is also seen in collaborative projects between regulatory agencies and industry. Notably, discussions within the ISAAA and national biosafety authorities are focusing on the development of digital sequence information (DSI) repositories, which will be critical for tracking and auditing combinatorial constructs as they enter commercial supply chains. These repositories are expected to become operational or enter pilot phases in 2025-2026, providing a backbone for traceability and compliance.
Looking ahead, the next few years will likely see convergence between regulatory policy, technical standards, and practical certification pathways. This includes the anticipated roll-out of internationally recognized labeling and data exchange protocols for synthetic biology products, enabling more predictable and transparent oversight of biocombinatorial junctions. As these frameworks solidify, industry participants and researchers will benefit from clearer guidance for product development and market entry, while regulators gain enhanced tools for risk management and public assurance.
Investment, Funding, and M&A Activity in 2025
Biocombinatorial junction engineering, at the intersection of synthetic biology and materials science, is experiencing a significant surge in investment and corporate activity as we enter 2025. This field, which focuses on the design and assembly of biological components to create novel junctions with tailored properties, is drawing heightened interest due to its applications in biotechnology, tissue engineering, and sustainable materials.
Notably, several synthetic biology leaders have announced expanded funding rounds and new partnerships targeting advanced junction engineering platforms. For instance, Ginkgo Bioworks has allocated substantial resources to its cell programming foundry, with a portion earmarked for projects involving the assembly of multi-domain protein junctions and biohybrid materials. In Q1 2025, Ginkgo’s strategic investment arm also participated in a $100 million Series C round for a startup specializing in programmable adhesion proteins for tissue scaffolding.
On the mergers and acquisitions front, Amyris, Inc. has made headlines by acquiring a niche biodesign firm with proprietary algorithms for combinatorial junction optimization. This move is expected to accelerate Amyris’ development of next-generation biobased polymers and composite biomaterials. Similarly, Twist Bioscience has entered into a strategic collaboration with a leading academic medical center to co-develop synthetic junction libraries aimed at regenerative medicine applications, providing both funding and access to its high-throughput DNA synthesis platforms.
Major life sciences investors are also increasing their focus on this domain. The corporate venture arm of Thermo Fisher Scientific recently announced a dedicated fund for enabling technologies in synthetic biology, with a priority on scalable engineering of biocombinatorial junctions for diagnostics and controlled drug delivery. Early-stage funding from such industry leaders is expected to spur further innovation and startup formation throughout 2025 and beyond.
Looking ahead, the outlook for biocombinatorial junction engineering remains robust. As regulatory clarity improves and proof-of-concept projects mature, additional M&A activity and late-stage funding rounds are anticipated, particularly from established biotech firms seeking to expand their portfolios in advanced biofabrication and smart biomaterials. Industry observers expect the ecosystem to see increased cross-sector partnerships, especially with companies in biomanufacturing, medical devices, and green chemistry, as the technology base broadens and commercial use-cases multiply.
Challenges & Risks: Technical, Ethical, and Commercial Barriers
Biocombinatorial junction engineering, which integrates biological, combinatorial, and materials science approaches to design and optimize molecular and cellular interfaces, is poised to redefine fields such as biosensing, regenerative medicine, and synthetic biology. However, as the field enters 2025, it faces a spectrum of challenges and risks across technical, ethical, and commercial domains.
- Technical Barriers: One of the foremost technical obstacles is the reproducibility and scalability of biocombinatorial junctions. The intricate assembly of biomolecules at engineered interfaces often results in variability between batches, impacting device performance and reliability. For instance, companies like Thermo Fisher Scientific and Merck KGaA have highlighted the need for robust, high-throughput screening and quality control for biofunctional materials. Additionally, the integration of engineered junctions with electronic or microfluidic systems remains a major engineering hurdle, as the biocompatibility and long-term stability of such interfaces are yet to reach industrial standards.
- Ethical and Regulatory Concerns: As biocombinatorial junctions increasingly involve synthetic biology and genetically modified components, ethical debates intensify around biosafety and potential environmental impacts. Regulatory frameworks, especially in the US and EU, are still adapting to these advancements. Organizations like the U.S. Food and Drug Administration and the European Commission Directorate-General for Health and Food Safety are actively reviewing protocols for pre-market approval and post-market surveillance of devices and therapeutics that utilize engineered biological junctions.
- Commercialization Hurdles: The translation from laboratory innovation to market-ready solutions is slowed by high development costs, uncertain intellectual property landscapes, and the need for rigorous validation. Companies such as Illumina, Inc. and QIAGEN, which are exploring advanced biointerface technologies, must navigate complex patent environments and demonstrate clear clinical or industrial value to attract investment and customer adoption. Moreover, the lack of standardized manufacturing guidelines impedes broad commercialization, making partnerships with established suppliers and contract manufacturers critical for scalability.
Looking forward to the next few years, the sector is expected to invest heavily in automation, quality assurance, and regulatory alignment. Advances in AI-driven design and microfabrication, as pursued by Thermo Fisher Scientific and Merck KGaA, may address technical reproducibility, while ongoing dialogue between industry and regulators will be pivotal for ethical and commercial progress.
2025–2030 Outlook: Future Opportunities and Transformational Trends
Biocombinatorial junction engineering, the rational design and assembly of interfaces between biomolecular components for advanced functionality, is poised for significant advances from 2025 to 2030. This field, situated at the crossroads of synthetic biology, protein engineering, and materials science, is expected to fuel breakthroughs in therapeutics, diagnostics, and sustainable manufacturing.
One of the primary drivers is the increasing precision of gene editing and protein design platforms. Companies such as Twist Bioscience and GenScript are expanding their capabilities in high-throughput, combinatorial synthesis of nucleic acids and peptides, enabling the rapid prototyping and screening of vast junction variants. By 2025, these advancements are expected to notably accelerate the optimization of enzyme cascades, biosensors, and smart biomaterials.
The integration of artificial intelligence and machine learning is another major trend. Platforms from IBM Research and open-source initiatives are being deployed to predict optimal sequence-function relationships at junction sites, automating the discovery of novel linkers and interfaces with desired mechanical, catalytic, or signaling properties. This computational-experimental synergy is projected to improve design success rates and shorten development cycles through 2030.
On the application front, biocombinatorial junction engineering is expected to transform next-generation cell therapies and biomanufacturing. For example, Amgen and Sartorius are investing in modular, programmable protein assemblies and synthetic scaffolds, aiming to enhance the specificity and efficacy of cell-based therapeutics and to create more efficient bioprocessing platforms. The ability to engineer precise molecular junctions will also unlock novel biosensor architectures, as seen in the ongoing development of multiplexed diagnostic platforms by Bio-Rad Laboratories.
Looking ahead, the maturation of microfluidic and automation systems—championed by companies like Fluidigm—will further scale up combinatorial screening and characterization of junction libraries. This will drive down costs and democratize access to high-complexity bioengineering projects, fostering more distributed innovation by startups and academic labs.
In summary, the 2025–2030 period is set to witness biocombinatorial junction engineering evolving from a specialized research niche into a mainstream technological paradigm. The convergence of digital design, synthetic biology, and automated screening is expected to yield functional biomolecular assemblies with unprecedented performance, catalyzing new solutions across medicine, industry, and environmental sustainability.
Sources & References
- Amyris
- Ginkgo Bioworks
- Integrated DNA Technologies (IDT)
- Twist Bioscience
- Novonesis (formerly Novozymes)
- Sangamo Therapeutics
- Synthego
- Evonik Industries
- Ginkgo Bioworks
- Thermo Fisher Scientific
- Ginkgo Bioworks
- Cognizant
- Thermo Fisher Scientific
- Synthetic Biology Engineering Research Center (SynBERC)
- Baxter International Inc.
- BASF SE
- imec
- International Service for the Acquisition of Agri-biotech Applications (ISAAA)
- European Commission Directorate-General for Health and Food Safety
- Illumina, Inc.
- QIAGEN
- IBM Research
- Sartorius